- Press Release
【Press Release】Successful optical biosensing using dual optical combs: High sensitivity and rapid detection of biomolecules with promising prospects
Key points
- Biosensing has been valuable for detecting biomolecules, including novel coronavirus (SARS-CoV-2), but achieving both high sensitivity and rapidity has been challenging.
- Rapid and high-sensitivity detection of SARS-CoV-2 was achieved by utilizing optical-to-electric frequency conversion of optical combs and active-dummy temperature compensation with dual-optical-comb configuration.
- This enables ultra-early detection of infectious pathogens, health biomarkers, food contaminants, environmental hormones, and more, contributing to various preventive measures.
Summary
Bio-sensors (Note 1) are biomolecular sensors that leverage or mimic the sophisticated molecular recognition capabilities of living organisms. They find widespread applications in fields such as healthcare, food, and the environment. In particular, optical biosensors, which optically read the interactions between target molecules and molecular recognition elements, offer high precision and rapid measurement capabilities. However, they have proven insufficient in terms of sensitivity for the ultra-early detection of novel coronavirus (SARS-CoV-2) and cancer cells.
The research group comprising Prof. Takeshi Yasui and Visiting Associate Professor Taira Kajisa of Institute of Post-LED Photonics, Tokushima University; Doctoral Course Student Shogo Miyamura of the Graduate School of Advanced Technology and Science, Tokushima University; and Associate Prof. Shuji Taue of the School of System Engineering, Kochi University of Technology has applied optical frequency comb (OFC) (Note 2), which have been gaining attention as the next-generation lasers, not as light sources but as sensors for biosensing(Note 3). In this research, we utilized the unique optical-to-electrical frequency conversion properties of OFC in order to address the aforementioned challenges, enabling sensing in the optical frequency domain and reading out in the electrical frequency domain. Furthermore, by employing twin OFCs (dual OFC) and implementing active-dummy temperature compensation(Note 4), we successfully mitigated the common issue of temperature drift(Note 5) in biosensing.
This breakthrough has enabled rapid and highly sensitive detection of SARS-CoV-2 and holds promise for ultras-early detection of emerging and re-emerging infectious viruses, as well as health biomarkers including cancer and environmental contaminants that pose health risks.
Research Introduction Video on YouTube by Prof. Yasui Lab. (Tokushima University)
https://youtu.be/j2crXxxmAyg
Research background and history
Biosensors capable of detecting various biological molecules of natural origin, such as sugars, nucleic acids, and proteins, leverage or mimic the intricate molecular recognition functions of living organisms. These biomolecular sensors find applications in a wide range of fields, including healthcare, food, and the environment. In particular, optical biosensors that read the interactions between target molecules and molecular recognition elements optically offer features such as high sensitivity, high precision, and rapid measurements. However, there is an ongoing need for further improvement in sensitivity. It is believed that significant enhancement of sensitivity in optical biosensors could enable ultra-early detection of novel coronavirus (SARS-CoV-2) and cancer. To achieve a substantial improvement in sensitivity, the introduction of a fundamentally different technological approach is essential.
In optical biosensing, measurements are based on the sample-concentration-dependent optical spectrum shift. However, the shift is relatively small compared to the width of the optical spectrum, and hence the performance limitations of optical spectrum measurement devices have constrained the sensitivity of biosensing. If it were possible to convert the sample-concentration-dependent optical spectrum shift into the corresponding electrical spectrum shift for measurement, it would be feasible to achieve high sensitivity in the optical domain while providing easy handling and high-precision measurements with electrical signals. Therefore, we have turned our attention to optical frequency comb (OFC) as a means of converting optical spectrum shifts into electrical spectrum shifts. By utilizing OFC, we can accurately convert optical frequency signals of 1014 Hz into electrical frequency signals of 108 Hz, making it possible to perform electrical frequency signal readout-based optical biosensing using high-performance and cost-effective electrical frequency measurement equipment. As a result, we are conducting research on biosensing with OFC as the core technology, which we believe holds great promise for advancing biosensing capabilities.
Research content and results
An OFC exhibits a highly discrete multispectral structure in which numerous optical frequency modes (OFC modes) are evenly spaced like the teeth of a comb (Fig. 1, upper part). The optical frequency νm of each OFC mode can be expressed by the following equation:
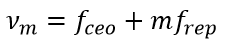
where fceo represents the carrier-envelope-offset frequency, frep is the mode spacing, and m is the mode order. While νm is an optical frequency signal of 1014 Hz, frep and fceo are electrical frequency signals of 108 Hz. This equation signifies the connection between optical and electrical frequency signals through the OFC. For example, by phase-locking frep and fceo to an electrical frequency standard through laser control, the uncertainty of the electrical frequency standard can be transferred to νm, allowing the OFC to serve as “optical frequency ruler” secured by the electric frequency standard (Fig. 1, bottom left). Conversely, this relationship can be utilized to convert optical frequency signals into electrical frequency signals. For instance, placing a fiber biosensor within the resonator of a fiber OFC enables the conversion of sample-concentration-dependent optical frequency spectrum shifts (νm shift) into electrical frequency spectrum shifts (frep shift) (Fig. 1, bottom right). Electrical frequency signal benefits from the availability of highly accurate national standards and the existence of high-performance, cost-effective measurement equipment, enabling high-precision, wide-dynamic-range, fast, and convenient measurements. By leveraging the excellent synergy between “optical-to-electrical frequency conversion in OFC ” and “high-precision electrical frequency measurement,” we have successfully enabled electrical frequency readout-based optical biosensing (biosensing OFC). This novel approach combines the high sensitivity of the optical frequency domain with the ease of handling and precision of electrical frequency readouts.
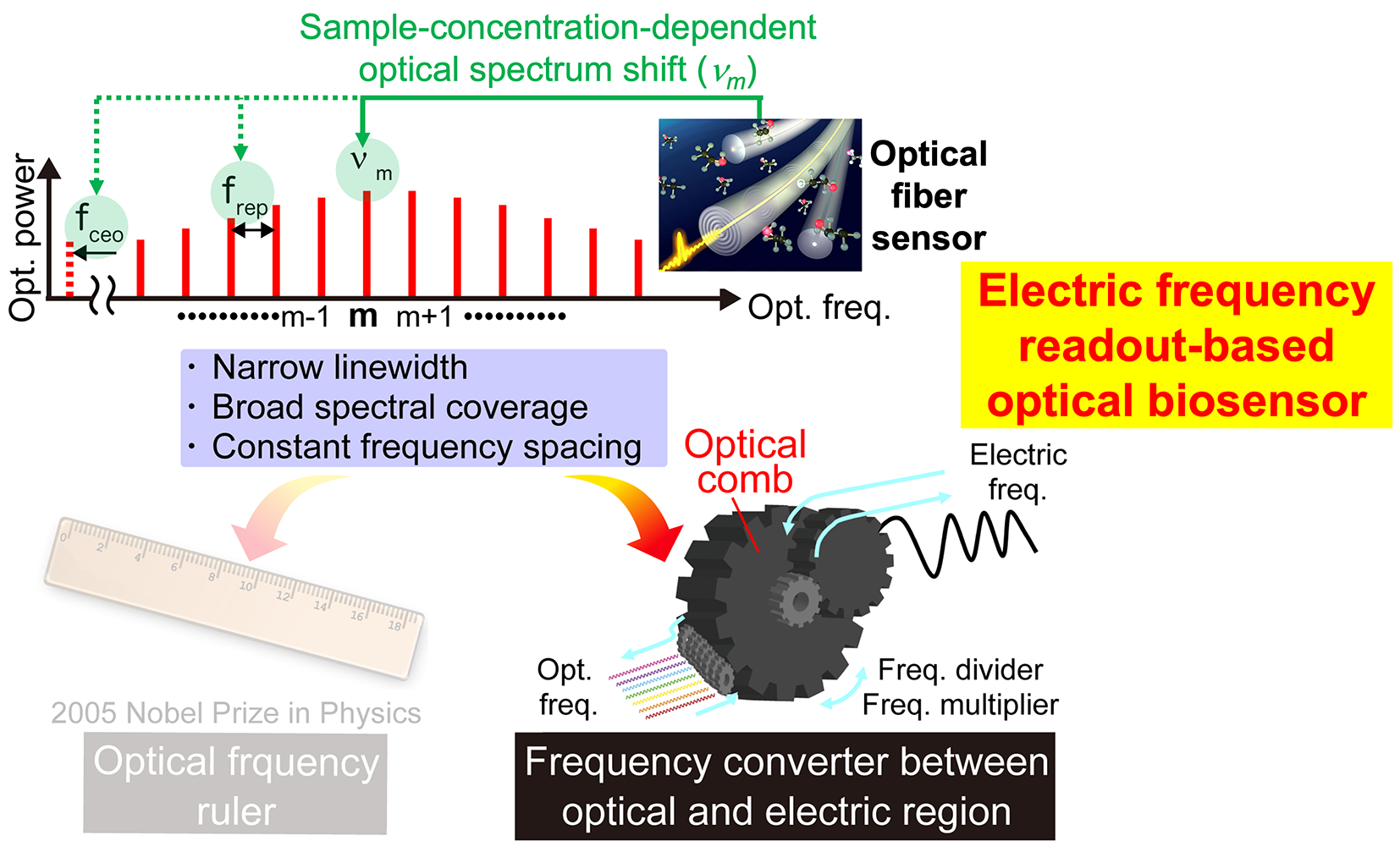
One of the remaining challenges in biosensing OFC is the temperature drift in the sensor signal of frep. frep is determined by the reciprocal of the optical resonator length of the fiber OFC, and it varies due to changes in environmental temperature, which cause thermal expansion and contraction of the optical resonator length. This temperature-induced variation in frep is superimposed on the sensor signal as temperature drift. Since the frep variation caused by temperature drift is often larger than the frep shift due to changes in sample concentration, an active-dummy temperature compensation approach has been employed (Fig. 2). In this method, equivalent fiber OFC resonators are prepared in pairs (dual OFC). One of the cavities (active-sensing OFC, mode spacing = frep1) incorporates a fiber sensor with surface modifications for biosensing (active sensor), while the other cavity (dummy-sensing OFC, ,mode spacing = frep2) remains another fiber sensor without surface modifications (dummy sensor). As both sensors are placed in the same sample cell, only the active sensor is sensitive to the antigen, while the effect of temperature drift is observed similarly in both sensors. Therefore, by measuring the differential signal between the active-sensing OFC and the dummy-sensing OFC (∆frep = frep1 – frep2), it becomes possible to compensate for the influence of temperature drift and extract only the signal dependent on sample concentration. This innovative approach significantly improves the accuracy and reliability of the biosensing OFC, allowing for precise and real-time detection of biological molecules while mitigating the effects of temperature fluctuations.
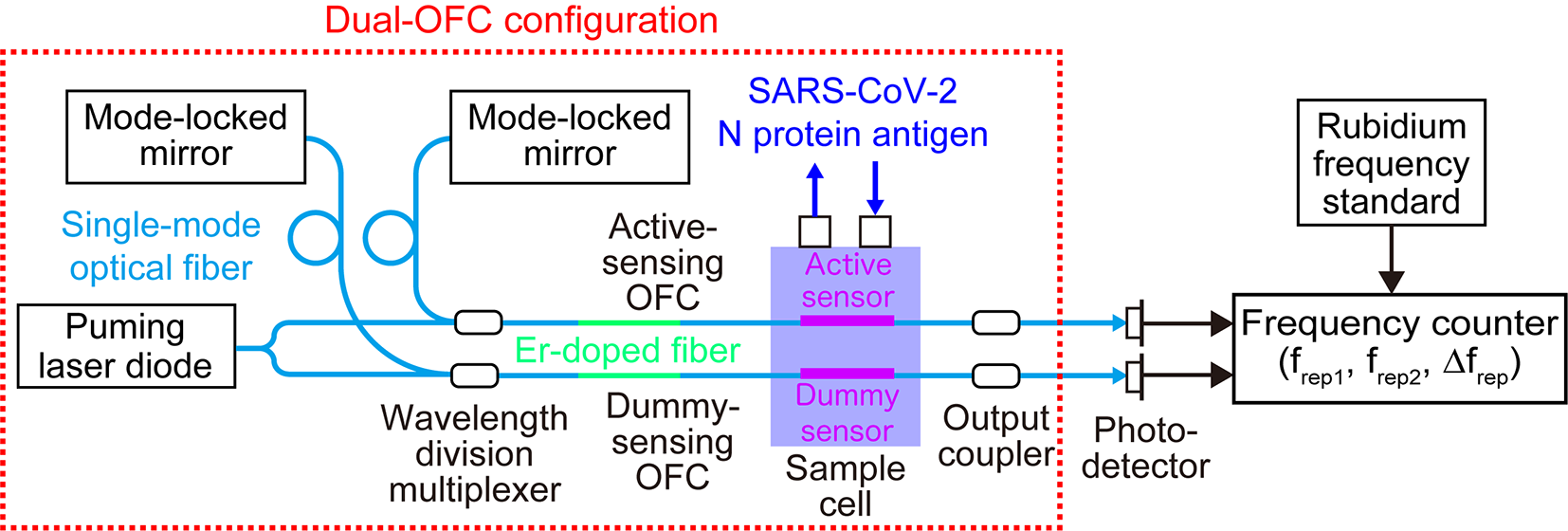
In this experiment, we conducted measurements while incrementally varying the concentration of the SARS-CoV-2 nucleocapsid (N) protein antigen in phosphate-buffered saline (PBS) at 10-minute intervals. Figure 3(a) illustrates the changes in sensor signals (frep1 and frep2) from the active-sensing OFC and the dummy-sensing OFC. In both sensor signals, a gradual change due to temperature drift is observed, but there is no evidence of step-wise changes related to antigen concentration in the active-sensing OFC signal. This is attributed to the fact that the temperature-induced variations in frep are more significant than the frep shifts caused by changes in antigen concentration. Then, the extracted differential signal (∆frep) between the two sensors is presented in Fig. 3(b). The temperature drift is effectively suppressed, and the step-wise changes corresponding to antigen concentration are clearly evident. From the measurement results, we generated a graph illustrating the relationship between antigen molar concentration and sensor signal, as shown by the red circle plots in Fig. 3(c). To assess the validity of the experimental results, we performed curve fitting analysis using a theoretical model (purple line). The analysis confirmed that within a 10-minute measurement time, the detection limit for molar concentration is 37aM. This rigorous validation underscores the accuracy and reliability of our biosensing approach and its remarkable sensitivity in detecting extremely low concentrations of the SARS-CoV-2 N protein antigen.
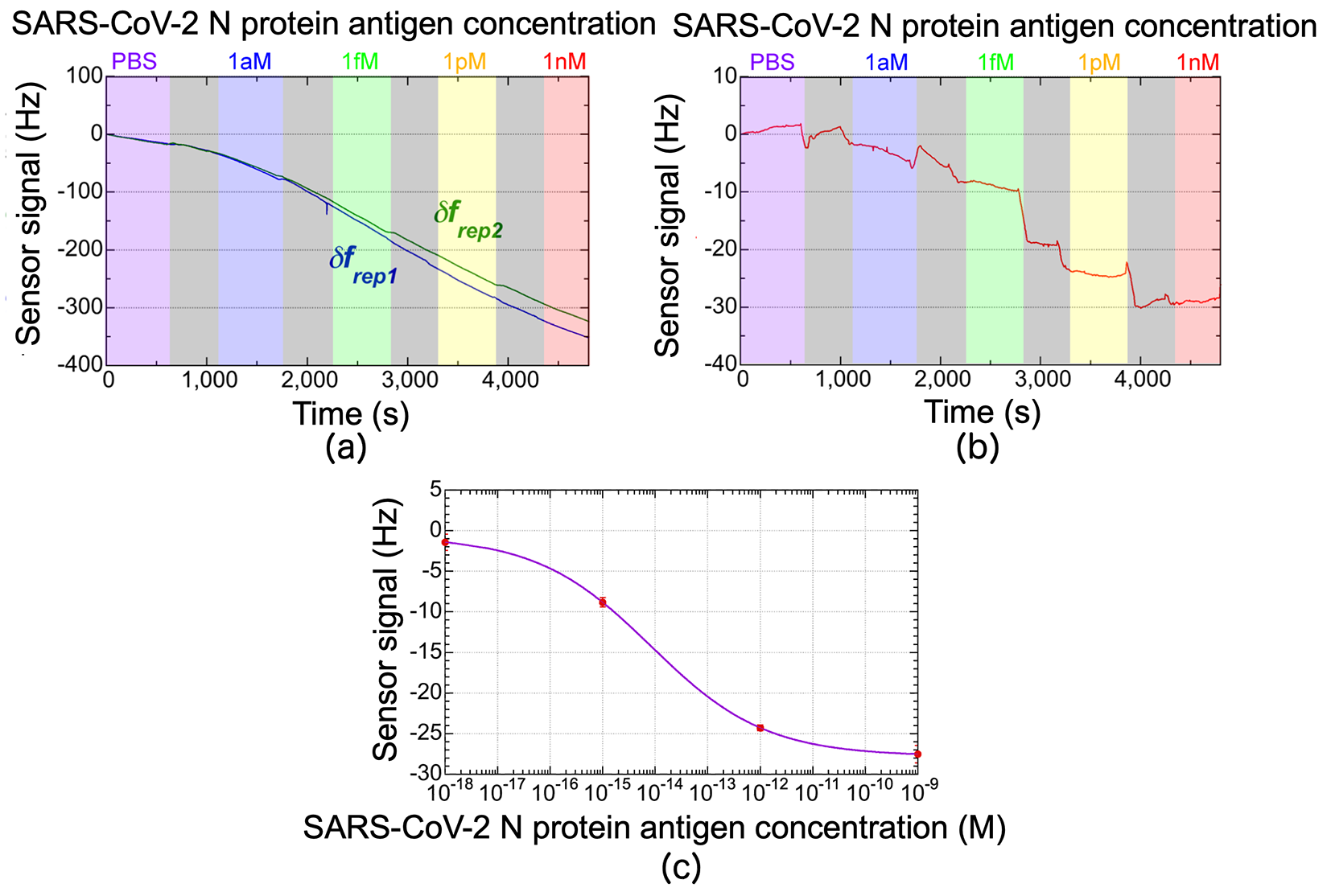
Division of roles
- Tokushima University: System design and construction, demonstration experiment.
- Kochi University of Technology: Fiber sensor design.
Future developments
While this measurement was conducted under ideal conditions with only the SARS-CoV-2 protein antigen present in PBS, real-world samples (such as saliva, nasopharyngeal swabs, and nasal swabs) often contain a multitude of unwanted proteins (interfering substances) due to non-specific adsorption. This non-specific adsorption can limit sensitivity and precision. In our future work, we plan to consider actual samples and implement sensor surface modification methods that prevent non-specific adsorption. This will allow us to assess the performance under conditions that closely resemble real-world scenarios and ensure the accuracy and reliability of our biosensing technology for practical applications.
Terminology
(Note 1) Biosensor
A biosensor is a device or sensor that detects biological or biochemical processes and converts them into electrical signals, optical signals, or other forms of signals. Typically, it involves immobilizing bio-derived molecules (such as enzymes or antibodies) on the sensor surface and detecting changes when target molecules bind to them. Biosensors are known for their high sensitivity and rapid measurement capabilities, making them valuable tools for applications ranging from monitoring blood glucose levels and detecting proteins to assessing environmental and food safety, identifying harmful substances and bacteria, and more recently, detecting novel coronavirus. They find applications in health and disease management, environmental monitoring, public health, and various fields.
(Note 2) Optical frequency comb (OFC)
OFC is a discrete multi-spectral structure comprising narrow-linewidth laser light (optical frequency modes) that can range from tens of thousands to several hundred thousand modes, uniformly spaced like the teeth of a comb (as shown in the upper section of Fig. 1). Through frequency stabilization control, it is possible to precisely determine the absolute frequencies of all the optical frequency modes that constitute the OFC. Consequently, OFC has been employed as “optical frequency rulers” in applications like gas spectroscopy analysis. In this context, our novel approach utilizes the OFC as an “optical-to-electrical frequency converter.” This opens up new avenues for applying OFC in the field of biosensing.
(Note 3) Biosensing
Biosensing refers to a set of techniques, methods, or approaches used to detect, monitor, or measure biological or biochemical processes using biosensors. Biosensing encompasses the entire process of utilizing biosensors to collect and interpret data.
(Note 4) Active-dummy temperature compensation
Active-dummy temperature compensation is a commonly used technique for compensating temperature effects in strain gauges (strain sensors). Strain gauges are sensitive to both strain and temperature, making it challenging to accurately measure strain in environments with temperature variations. To address this issue, an equivalent pair of strain gauges is prepared. One strain gauge is attached to the site where strain is measured (active strain gauge), while the other is placed nearby in a location where strain does not occur, but temperature changes are expected to be similar (dummy strain gauge). While the active strain gauge measures both strain and temperature changes, the dummy strain gauge only measures temperature changes. By calculating the difference signal between the two gauges using a bridge circuit, the influence of temperature changes is effectively canceled out, allowing for the measurement of strain alone, as illustrated in Fig. A.
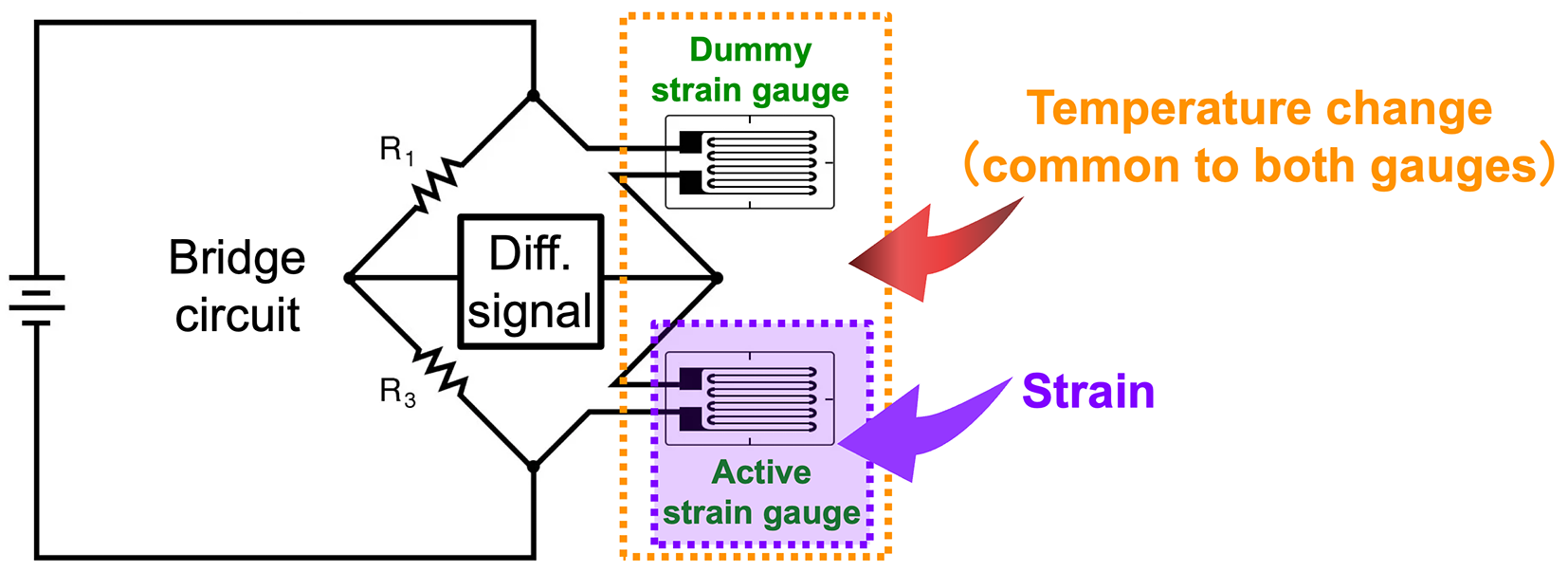
(Note 5) Temperature drift
In biosensing, temperature drift refers to the phenomenon where changes in temperature, either in the biosensor itself or in the biological sample, can influence the measurement results of the biosensor. Temperature drift is an important factor that needs to be taken into consideration when biosensors are used to perform accurate measurements.
Acknowledgments
We acknowledge the financial support for the project from Cabinet Office, Government of Japan (Promotion of Regional Industries and Universities) as well as from the Japan Society for the Promotion of Science (22H00303), Japan Agency for Medical Research and Development (20he0822006j00), Japan Science and Technology Agency (JPMJMS2025), Tokushima Prefecture (Creation and Application of Next-Generation Photonics), and Research Clusters program of Tokushima University (2201001).
Reference
Journal | Scientific Reports |
Title of original paper | Rapid, high-sensitivity detection of biomolecules using dual-comb biosensing |
Authors | Shogo Miyamura, Ryo Oe, Takuya Nakahara, Hidenori Koresawa, Shota Okada, Shuji Taue, Yu Tokizane, Takeo Minamikawa, Taka-Aki Yano, Kunihiro Otsuka, Ayuko Sakane, Takuya Sasaki, Koji Yasutomo, Taira Kajisa, and Takeshi Yasui |
DOI | 10.1038/s41598-023-41436-3 |
Related patents
We have filed three related Japanese patent applications.